Ultrafast spectroscopy
Through understanding and controlling the dynamics in complex condensed matter systems, ultrafast spectroscopy is being used to provide insights that may enable future low-energy electronics technologies and efficient solar energy solutions.
Using pulses of light that are a millionth of a billionth of a second long, this research program strives to understand and control the dynamics and mechanisms at play in complex condensed matter systems. The development of new approaches for ultrafast spectroscopy allows us to push the limits of what can be revealed and understood in complex material systems across physics, chemistry and biology.
From novel quantum materials that may be the basis for future low-energy electronics technologies, to the long-standing problems of understanding the mechanisms of high-temperature superconductivity and photosynthesis, ultrafast spectroscopy is providing new insights across a range of materials that have the potential to play a major part in future technologies.
Our research projects
Floquet engineering of the bandstructure in 2D materials
The advent of 2D materials, which began with the discovery of graphene, has opened the door to a vast range of new physics and novel quantum phases of matter. Combining these materials with Floquet engineering, which uses a periodic driving field to alter the bandstucture, it then becomes possible to control and switch the properties of the materials.
We're using femtosecond laser pulses to drive the Floquet-Bloch states in 2D transition metal dichalcogenides and graphene. We can probe these systems with subsequent femtosecond pulses, including making use of our multidimensional coherent spectroscopy to follow the coherent dynamics through the switching on/off of the Floquet states.
Amongst the new phases of matter with significant potential for applications in future low-energy electronics technologies are 2D topological insulators. These materials are insulating in the bulk but can sustain current flows around the edges without resistance. We are extending the ideas of Floquet engineering in 2D materials to drive a topological phase transition — that is, to drive a 2D material from being a trivial insulator into a topological insulator or vice versa. This type of phase transition will be important for the potential application of topological insulators in future low-energy electronics technologies and by exploring these process on an ultrafast timescale we will develop the understanding that can make this a reality.
2D materials and their heterostructures
Since the Nobel prize-winning discovery of graphene (a 2D sheet of carbon atoms, 1 atom thick) in 2006, there has been rapid growth in research on graphene and other 2D materials with remarkable and diverse properties. There is much that is still unknown in many of these materials and we are using femtosecond lasers and ultrafast spectroscopy techniques to reveal details of the interactions between electrons, holes, excitons and the underlying band structure in these novel 2D materials.
By stacking different 2D materials it is possible to realise vastly different properties. For example, two layers of graphene stacked at the right angle can become a superconductor. With the growing array of 2D materials available, new combinations are frequently revealing new physics. We’re focused on excitonic materials and the potential of inter-layer excitons to form a superfluid that could lead to charge flow without resistance and the potential for future low-energy electronics technologies.
We are using our array of ultrafast spectroscopy and microscopy techniques to understand these materials, how we can control the coupling between layers and how this impacts on the dynamics of the resultant excitons. This in turn will help realise new ways of utilising these 2D material systems for future device applications.
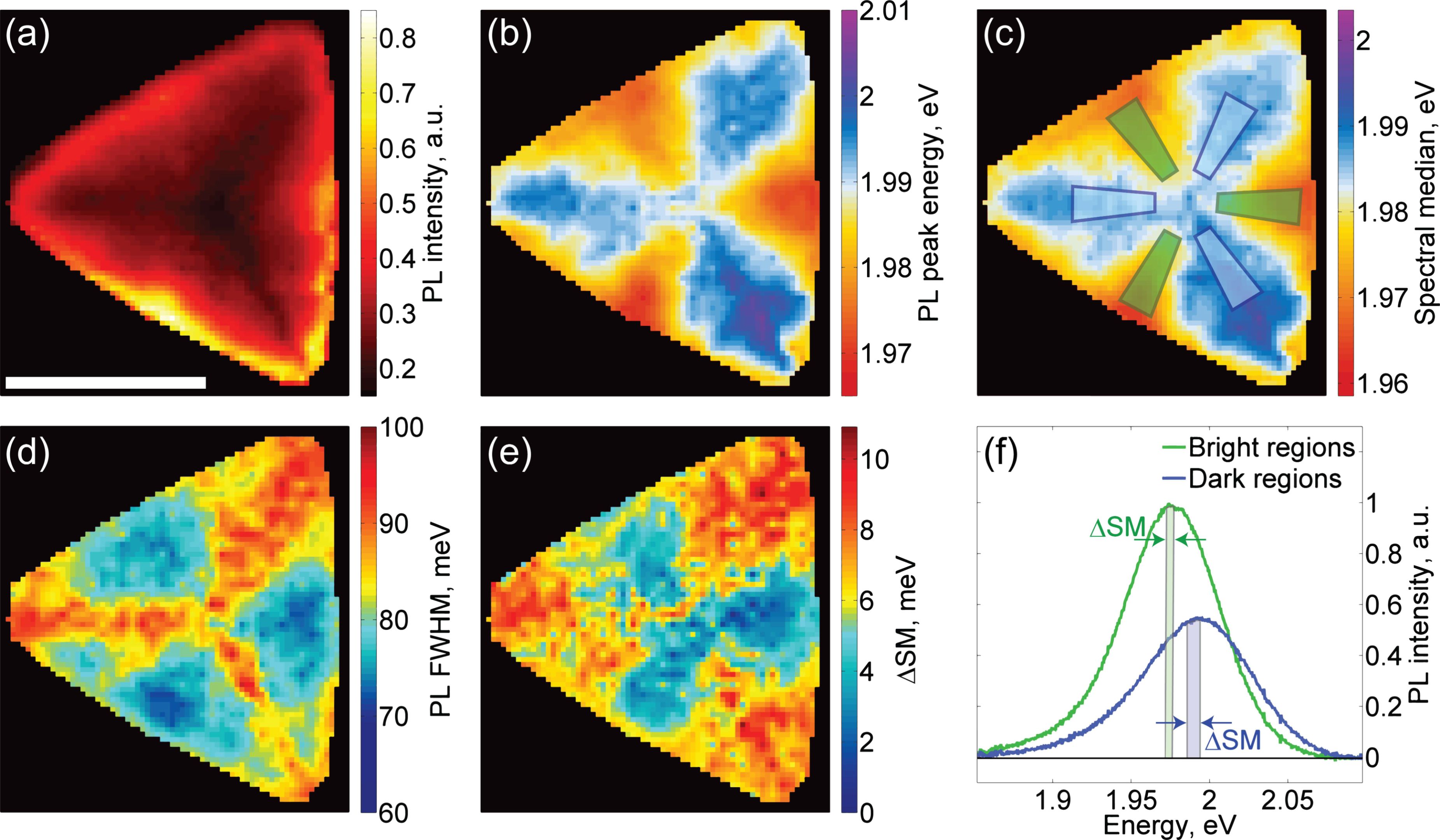
Atomically thin two-dimensional semiconductors (like the WS2 shown here) possess many interesting and potentially useful properties, which we are exploring and seeking to control. Here we map the spectral properties from a CVD grown flake of WS2. [2D Materials 7, 025008 (2019)].
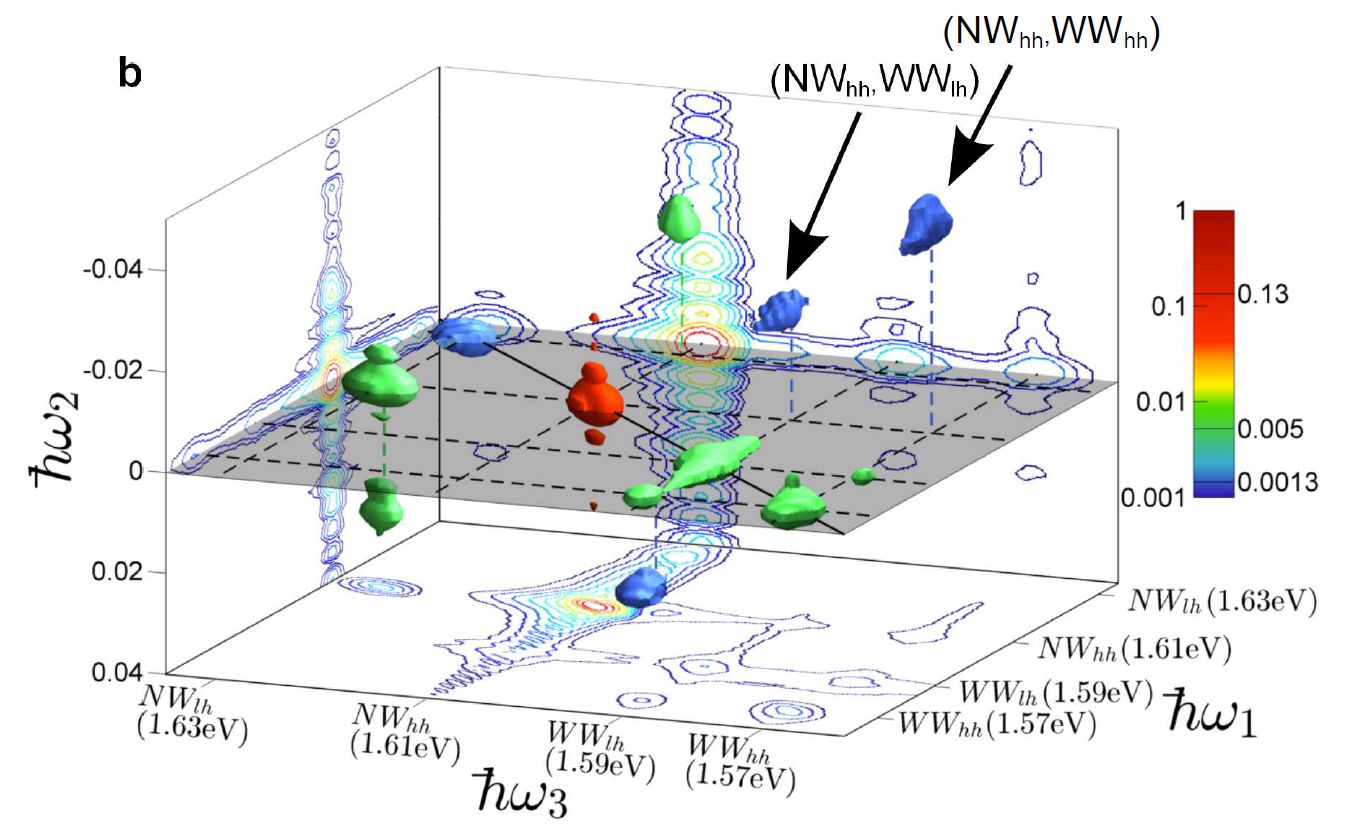
Multi-Dimensional Coherent Spectroscopy allows interactions between states to be identified and quantified. This three-dimensional (3D) spectrum reveal coherent coupling between excitons in distinct quantum wells. [Progress in Quantum Electronics 55, 1-34].
Strongly correlated materials
Strongly correlated electron materials are characterised by emergent collective behaviour that can lead to vastly different macroscopic properties, including high-temperature superconductivity, heavy Fermion behaviour, multiferroicity and more. The prospect of controlling these materials and their properties by design of the structure, or by active control, is an exciting one that may lead to the realisation of new quantum phases of matter and/or novel device applications. However, the requisite detailed understanding of the origin of these emergent collective behaviours is missing and represents one of the greatest unsolved problems in physics today.
In each of these symmetry-breaking, ordered phases the bosonic modes (i.e. fluctuations of lattice (phonons), spin or charge order) shape the potentials by which the electrons interact. Understanding the nature of these bosonic excitations in different phases, how they interact with each other and how they mediate the electron-electron interactions is key to predicting and ultimately controlling the macroscopic behaviour that emerges from the underlying microscopic structure of strongly correlated materials.
We are using Multidimensional Coherent Spectroscopy to identify signatures of specific low-energy excitations in different phases of prototypical strongly correlated materials. Based on this knowledge, we aim to disentangle the nature and role of different low-energy excitations and the effects of coherence in materials where the interactions are crucial, including high-temperature superconductors.
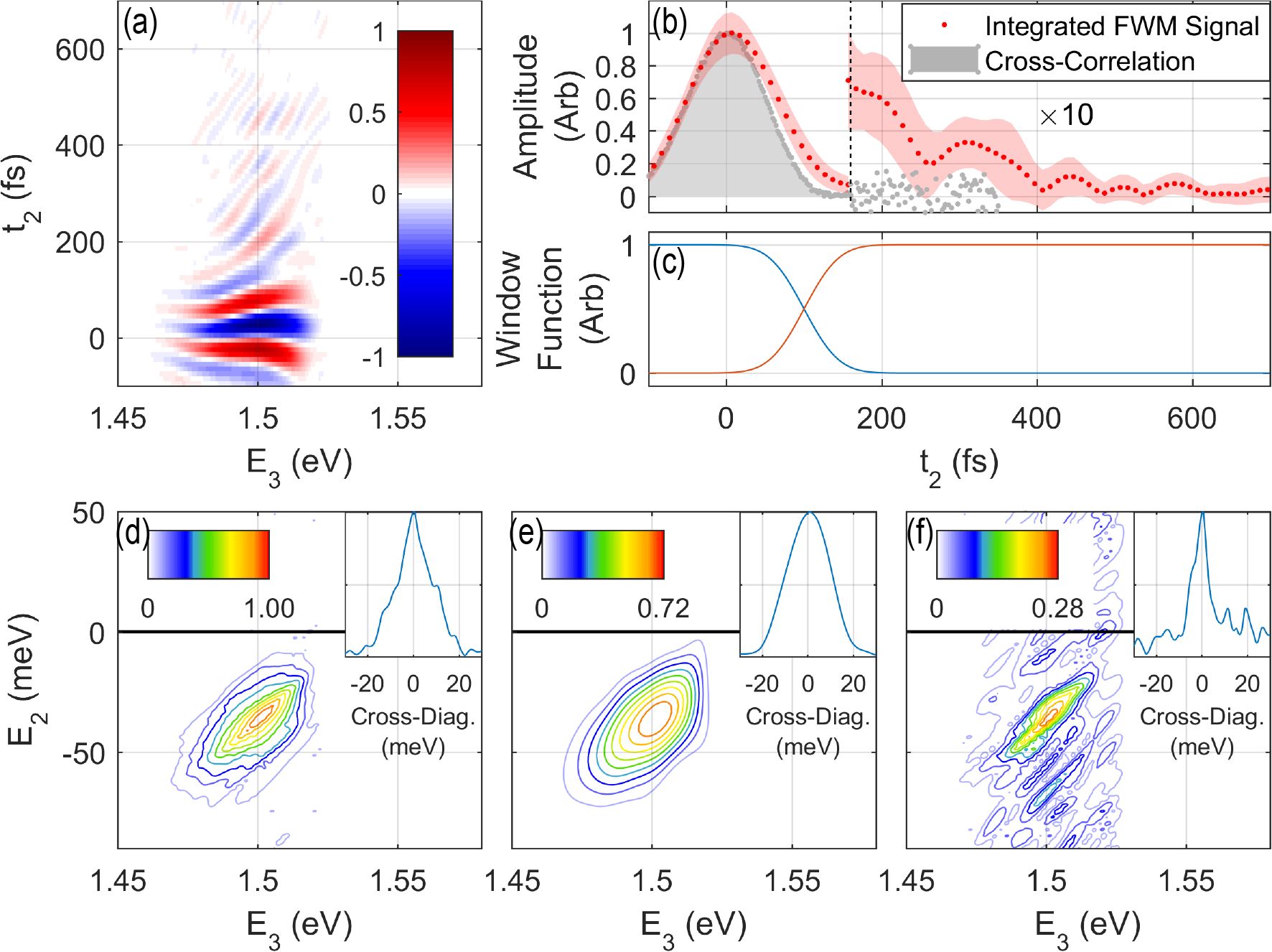
Pathway selective coherent spectroscopy reveals persistent coherences that in turn identify correlations between low-energy modes and optical transitions in a high-temperature superconductor. [Science Advances 6, eaaw9932].
Quantifying interactions in exciton polariton systems
A polariton is a quasi-particle that is part light and part matter. We’re looking at exciton polaritons where the matter part is an exciton in a semiconductor material (quantum well or 2D material) within a microcavity, which confines the light. The interactions between polaritons and between polaritons and excitons play an important role in determining the macroscopic properties and ability to realise quantum phases of matter (such as Bose-Einstein condensates). Understanding these interactions and how they can be controlled is complicated and some questions remain unresolved. We are using multidimensional coherent spectroscopy to quantify these interactions with wavevector selectivity and understand how they vary and how they can be controlled.
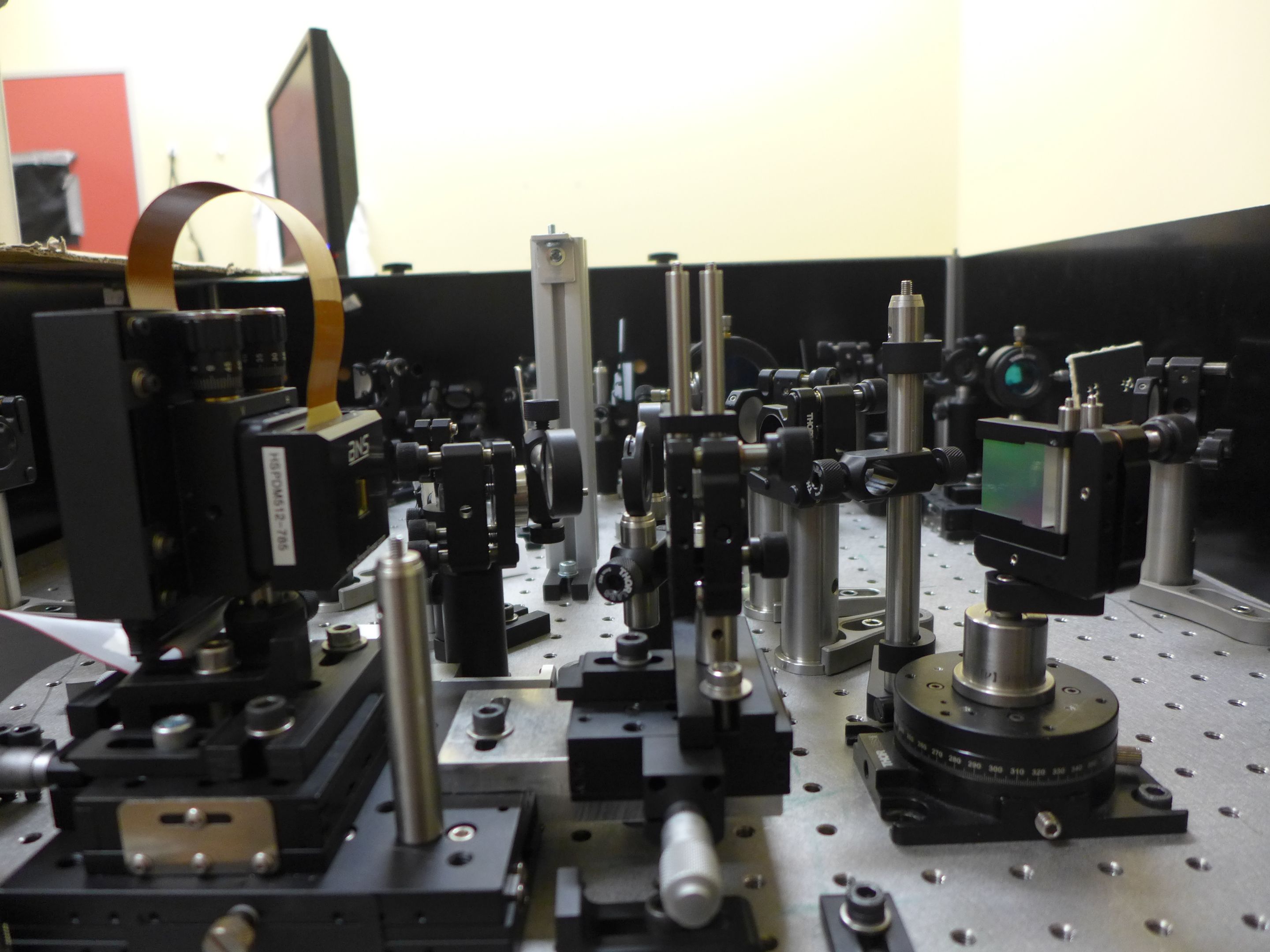
Multidimensional coherent spectroscopy setup, enabled by a pulse shaper based on a spatial light modulator.
Energy transfer and coherent dynamics in photosynthesis
Photosynthesis is the process by which energy from the sun is absorbed — by plants, algae, some bacteria — and stored in chemical bonds. In the early stages, the energy is absorbed by chromophores in pigment protein complexes and then transferred to other chromophores and complexes before reaching the reaction centre, where all the biochemistry starts to happen. These energy transfer steps occur with very high quantum efficiency. Ultrafast spectroscopy has been crucial in revealing the dynamics of these ultrafast energy transfer processes, though the precise mechanisms of the different energy transfer processes remains a subject of some conjecture.
Recent work has revealed the important role of vibrations in mediating electronic energy transfer. As a result, understanding the interplay between the electronic and vibrational degrees of freedom has become crucial. We utilise our unique approach for multidimensional coherent spectroscopy, which allows pathway selectivity through spectral shaping to probe these interactions and measure the dynamics of energy transfer.
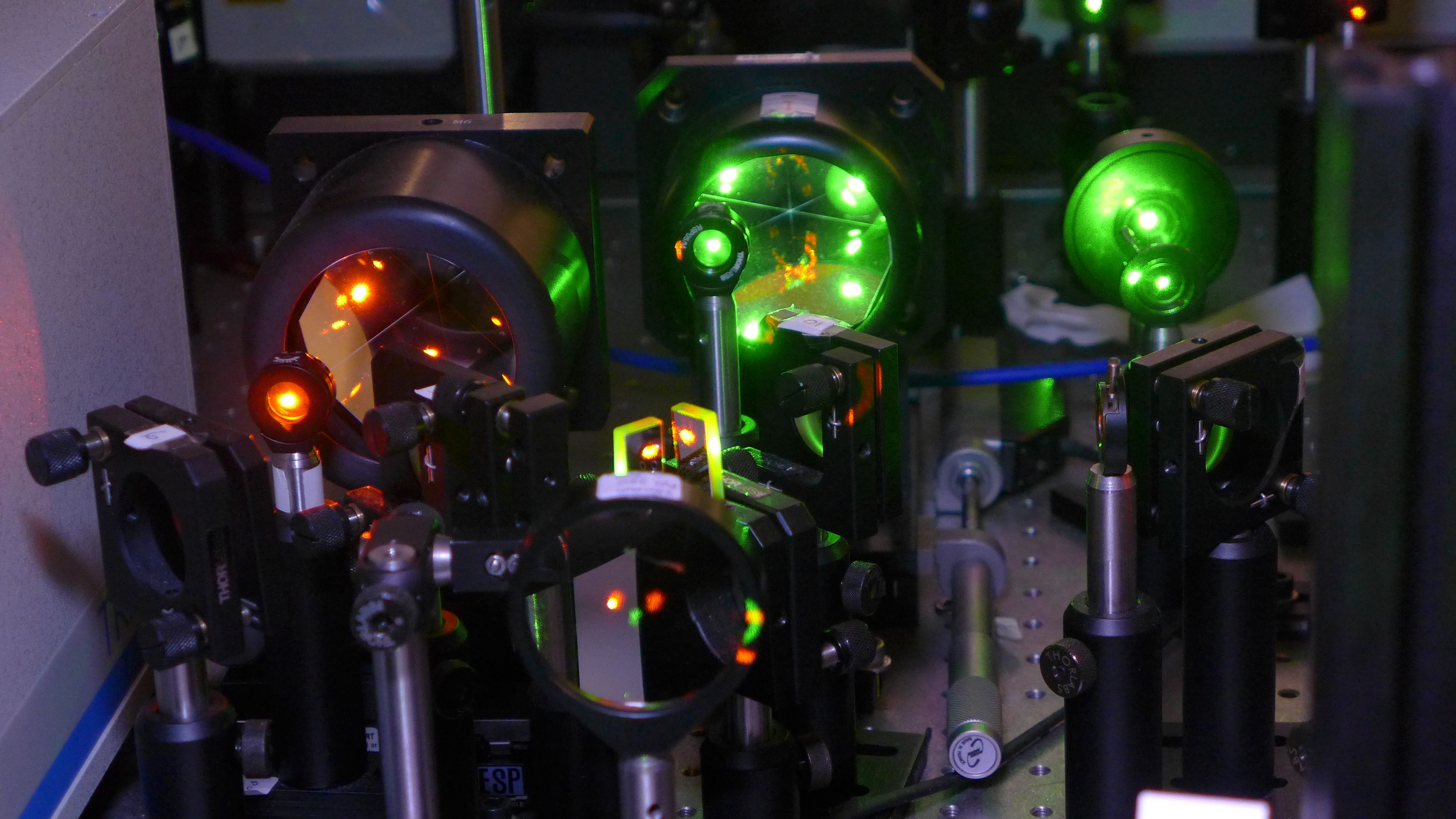
Two synchronised NOPAs (Non-colinear optical parametric amplifiers) allow us to perform two-colour experiments across the visible and near-IR, where we can identify coherent coupling, and energy transfer, and transiently control (and measure) the bandstructure of 2D materials.
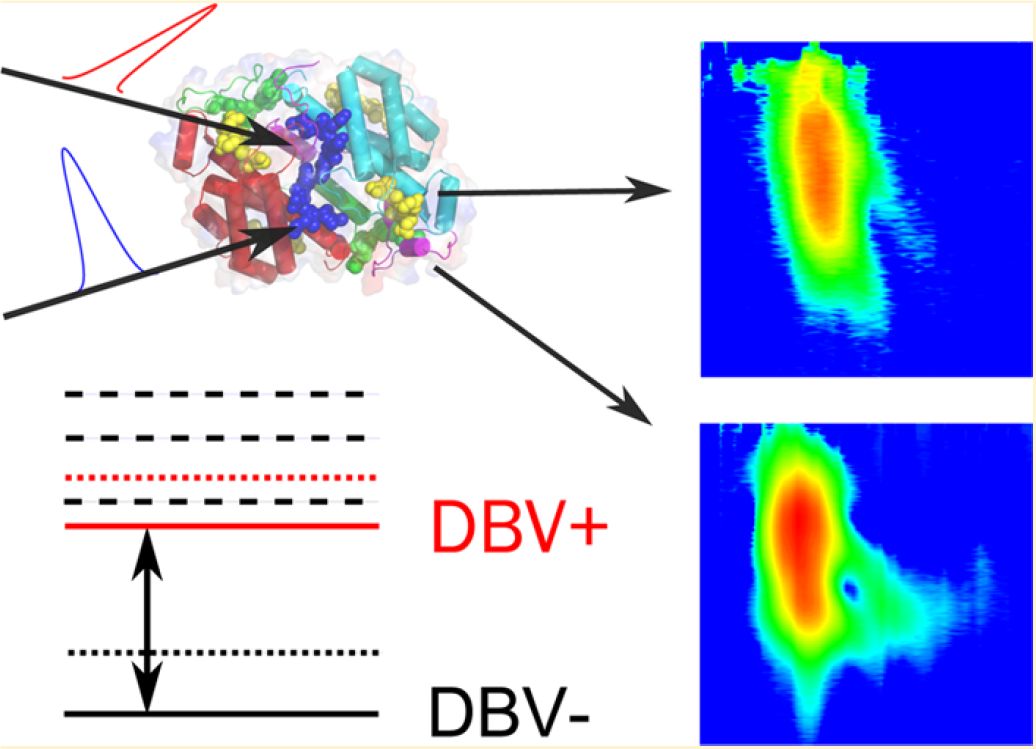
Two-colour coherent spectroscopy has allowed the identification of vibrational and vibronic coupling in photosynthetic light harvest complexes such as PC645 [J. Phys. Chem. Lett. 6, 4573 (2015)].
Our team
- Associate Professor Jeffrey Davis (team leader)
- Dr Jonathan Tollerud
- Dr Stuart Earl
- Tatek Lemma
- Rishabh Mishra
- Mitchell Conway
- Jack Muir
Keen to be involved with our research?
Honours and PhD projects are available associated with each of the research projects listed. Please contact Associate Professor Jeffrey Davis for further details or check out more project opportunities in other research areas.
Funding
ARC Centre of Excellence for Future Low-Energy Technologies
Explore our other research programs
-
Anyon technologies
-
Applied optics
-
Cell biophysics
-
Complex systems
-
Immune cell fate determination
-
Nonlinear physics
-
Optical nanoparticle spectroscopy for photonic application laboratory
-
Dysprosium quantum gas microscope laboratory
-
Quantum theory
-
Time crystals with ultracold atoms
-
Ultracold fermi gas laboratory
Contact the Optical Sciences Centre
There are many ways to engage with us. If your organisation is dealing with a complex problem, get in touch to discuss how we can work together to provide solutions. Call us on +61 3 9214 8096 or email osc@swinburne.edu.au.