Our research
Learn about the cutting-edge research currently being undertaken by the Fluid and Process Dynamics Research Group.
The group is focused on cutting-edge and high-impact research to transform industries in the areas of fluid dynamics/mechanics, fluid structures, thermodynamics, materials and energy transformation, and high temperature chemical and materials processes.
The knowledge and science in these sub-disciplines underpins the research undertaken by the majority of Swinburne’s research institutes, with expertise provided by the Fluid and Process Dynamics Research Group.
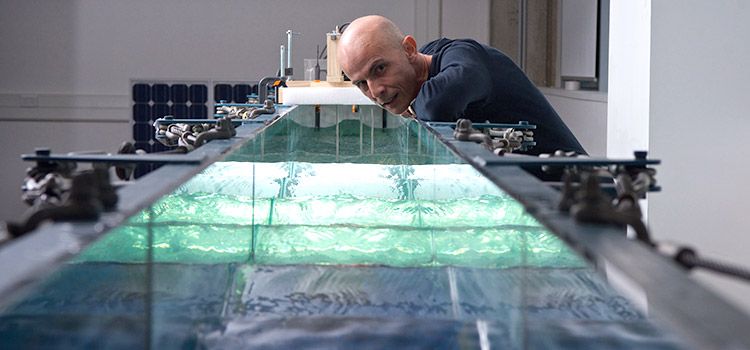
The study of bubble acoustics incorporates many interesting fluid-dynamical and mathematical problems, with fascinating real-world applications. Few people realise that much of the sound we associate with water in motion, from the plink of a raindrop hitting a pond to the roar of the surf, is due to the formation of bubbles. Bubbles have a natural frequency of volumetric oscillation, so they can effectively ring like bells. These sounds created by bubbles are exploited in industrial and environmental instrumentation.
Whenever gases are injected into liquids in industry, there is a need to measure the size of the bubbles formed. Whether it is the processing of minerals such as copper or the oxygenation of wastewater, bubble size matters. Often, measurement of the bubble-acoustic frequency is the only way to get these data. Bubble formation occurs in the ocean whenever waves break, and thus accelerates the dissolution of atmospheric carbon dioxide. Bubble-acoustic buoys are proposed to measure the rate of oceanic carbon dioxide absorption, a vital factor in the rate of global warming.
As well as making sounds, bubbles also respond to sound; intraveneously injected microbubbles are used in diagnostic medical ultrasound. Research is also underway into developing new targeted therapies for diseases such as cancer by causing bubbles to rupture in an ultrasound beam. Microbubbles can also enhance recently-discovered possible therapies such as the modulation of neural signals in the brain.
Contact: Professor Richard Manasseh. PhD topics in this area are available.
This research is focussed on the development of flow simulation software capable of simulating flows in and around structures that are free to move and deform. This is done using a sharp-interface immersed-boundary method, which reduces the complexity associated with mesh construction and deformation associated with more traditional simulation codes. It also means very large simulations can be conducted using high-performance computing facilities such as Swinburne's Supercomputer. The current implementation of this method has simulated flapping foil energy harvesters, the vibration of tube arrays, reciprocating flows through curved tubes and flapping fish fins.
Contact: Dr Justin Leontini
Fluids like wind and water flowing past structures are ubiquitous in engineering and nature. Fluid-structure interaction concerns situations where the dynamics of the flow and the structure are coupled and need to be treated together. Our research is focussed on understanding the fundamental phenomena involved in these situations, in particular those that cause a structure to shake or vibrate, including vortex-induced vibration, fluid-elastic galloping and aeroelastic flutter. Swinburne’s Supercomputer is being used to create simulations to untangle these complex and highly nonlinear phenomena.
Recent projects include:
- Vortex-induced vibration of a diamond cross-section
- Three-dimensional structure in the wake of an elliptic cross-section
- Asymetric vortex shedding behind an oscillating cylinder.
Contact: Dr Justin Leontini
Unlike wind energy, there is no single established concept for generating electricity from ocean waves. The inherently reciprocating (to-and-fro) nature of waves, in which the speed of the water is never steady and constantly reverses, demands a more complex generation mechanism.
Many types of ocean Wave Energy Converters (WECs) are being developed and trialled worldwide, including several in Australia. These machines convert the ocean swell into power, a source of reliable renewable energy with great value to future electricity markets. Swinburne’s wave-power research includes studies of fundamental fluid dynamics inspired by wave energy conversion, as well as studies of potential environmental impacts, and a project on wave energy converter arrays or ‘farms’.
An array of WECs could act together as one collective machine, with significantly different behaviour to a lone device. This collective behaviour is not well understood, and this project uses a combination of mathematical modelling and laboratory experiments to deliver the ability to better predict the performance of small arrays of WECs. This project is supported by a $770,000 grant from the Australian Renewable Energy Agency (ARENA), led by Swinburne in partnership with two Australian wave-power companies and the Australian Maritime College at the University of Tasmania.
Read more on our wave-power projects, and the ocean-related facilities within our Energy Transformation Laboratory. Via partnerships with other universities, Swinburne also has access to wind-wave and ice-wave channels and wave basins.
Contact: Professor Richard Manasseh
Our research is focussed on understanding the fundamental gas transport mechanisms present in high-frequency ventilation, with the aim of optimising the process and improving the clinical outcomes for patients. Often in intensive care units, patients require assisted ventilation to breath. For patients with damaged or delicate lungs, one strategy is using high-frequency ventilation, consisting of very fast, very small inflations. The small amount of inflation means the patient's lung tissue is not overly distended, protecting it from further damage. However if only small amounts of air are added and removed each breath, how is carbon dioxide removed and oxygen provided? If the lungs are not emptied and refilled each breath, how are gases transported?
Contact: Dr Justin Leontini
Understanding thermodynamics and phase equilibria provides the basis for improving existing and developing new mineral, metal and material processes. Our research spans different metals, oxides and sulphides systems and recent projects include:
- Thermodynamic behaviour of precious and rare metals (Ge, Te, Se, Pd) during secondary copper recycling
- Thermodynamic modelling of carbosulphidation of alumina
- Phase equilibria of Fe-Ni-O-X system relevant to nickel production from laterite
- Thermodynamic assessment of Fe-Cr-Ti-O-S system relevant to ilmenite processing.
Contact: Professor M Akbar Rhamdhani
The group focuses its research on various aspect of processing and recycling of urban ores (alternative metal sources) in support of resource efficiency and circular economy. The research projects are ranging from:
- Refining of Si and separation of Ag during recycling of solar panels (PV Cell)
- Recycling of Li-ion electric vehicles batteries through hydrogen reduction, carbothermic and aluminothermic reduction
- Development of a mobile e-waste processing facility
- Recycling of alkaline batteries using concentrated solar energy
- Processing of end-of-Life rare earth permanent magnet through oxidation.
Contact: Professor M Akbar Rhamdhani
Our research focuses on the use of concentrated solar energy to process minerals and make metals. Swinburne has built an in-house 42kW solar concentrator simulator that can reach in excess 1500 C.
Some of the current projects:
- Solar thermal processing of iron ores.
Contact: Professor Geoffrey Brooks
- Solar thermal processing of ilmenite ores.
Contact: Professor M Akbar Rhamdhani
The group also focuses on research on extraction of metals, minerals processing of extra-terrestrial resources. These include in-situ lunar and Mars resources utilisation.
Some of the current projects:
- Lunar mineral processing through thermal decomposition using concentrated solar energy
- Properties of icy lunar and Martian regolith simulants and their implications on In-Situ Resource Utilization.
Contact: Professor Geoffrey Brooks
- Fundamental of molten oxide electrolysis for lunar oxygen and metal production
- Thermodynamic Assessment of Mars mineral processing.
Contact: Professor M Akbar Rhamdhani
Our group uses advanced computation fluid dynamics (CFD) to understand a wide range of metallurgical processing/reaction systems, minerals processing and combustion processes. Some of the projects include:
- Numerical modelling of fluidized bed combustion
- Bubbles and foams behaviour in froth flotation
- Biomass combustion.
Contact: Associate Professor Jamal Naser
Contact the Fluid and Process Dynamics Research Group
If you want to know more about this research group or are interested in partnering with us, contact us on FPDResearch@swinburne.edu.au