Transforming quantum theories into quantum technologies
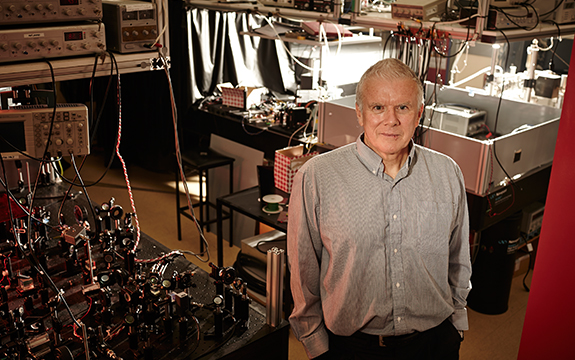
In Summary
-
This article featured in Swinburne’s new ‘Research Impact’ magazine, produced in association with Nature Publishing Group
Our world, as we commonly observe, is neatly governed by classical laws of physics — objects have mass and they move in predictable ways in responses to natural forces. Down at the sub-atomic level however, things become more nebulous, transitioning to the probabilistic world of quantum physics where the mere act of observing an object can change reality.
Professors Margaret Reid and Peter Drummond from Swinburne University of Technology lead parallel groups that are at the forefront of global research into understanding and translating some of the most exotic and peculiar quantum effects into practical technologies.
Ever since the early 20th century, in the era of Einstein, Schrödinger and Bohr, when the concepts of quantum uncertainty emerged, physicists have argued vigorously about the meaning, mechanism and implications of some of quantum physics’ most intriguing phenomena. Fundamental to quantum reality is the idea that objects classically thought of as particles, like electrons, occupy not a point but an envelope in space, a kind of ‘fuzzy’ localisation with the character of a wave. And in the same way that an object’s location may be fuzzy, so are its other properties, such as momentum and spin. Taking this a step further, quantum physics allows these wave–objects to be ‘split’ as a wave, resulting, for example, in one electron occupying two discrete regions of space, or two different energy levels or spin states. This ‘superposition’ of states persists until the object is measured or observed, which causes the superposition to collapse and the object to revert to its classical, definitive form.
As bizarre and exotic as it may sound, quantum superposition is now generally accepted as fact and experimentally observable.
For example, physicists 20 years ago demonstrated that it’s possible to ‘split’ a stream of sodium atoms such that each atom travels two paths simultaneously, ultimately producing a self-interference pattern as evidence of the fact when the paths are recombined. So far whole atoms are the largest objects that have been put into such superposed states, but science is on the cusp of pushing the quantum-to-classical transition into the realm of larger objects to produce what quantum physicists call a Schrödinger cat — a macroscopic object like Schrödinger’s theoretical cat that is both alive and dead until the box in which it sits is peered into.
Another implication of the quantum universe is the ability for two particles, often photons, to ‘entangle’. In this scenario, the particles’ positions and momenta are correlated, with the momentum of one particle being the inverse of the other. The two entangled particles maintain their correlated states of fuzzy superposition until measured, but once one of the pair is observed, the state of the other is influenced instantly, regardless of distance separating them. Since the measurement of one particle’s position, momentum or spin appears to change the properties of the other, physicists refer to this behaviour as steering. Einstein called it ‘spooky’ action at a distance, and the nature of it is at the crux of current quantum physical debate.
Quantum technology
Far from being an esoteric theory limited to the subatomic domain, quantum physics has some very exciting and potentially revolutionary real-world applications. Quantum computing, which makes use of the fuzzy superposition of states to allow many calculations to be performed simultaneously, has been the vanguard for quantum technology. But such devices have very specific, niche applications like solving complex optimisation problems and simulation of quantum systems and so are unlikely to replace your laptop computer any time soon. Yet there are potentially more useful applications under investigation, most of which rely on entanglement for measurement, communication and security.
Reid’s group is applying spooky entanglement for secure communications using quantum cryptography. “We know entanglement can be used for quantum cryptography using standard existing cryptography schemes,” she says. While traditional digital encryption systems rely on two users exchanging a key, often a large number, to establish a secure communication channel, quantum cryptography encodes the key in the spin states of quantum bits — the basic data unit used in quantum computing and the equivalent of the 1s and 0s used in conventional digital computing. “For example, two parties can share a spin entangled pair of particles. By exchanging a choice of measurement variable via a public channel, it is possible to verify that they share the same bit value, forming a key that they can then use to read the encrypted message.”
If an eavesdropper attempts to intervene and measure the bit values, this shows up as a loss of entanglement, since in quantum mechanics, a system cannot be measured without changing it. The advantage is that due to physical laws one can be certain that a message has not been intercepted, making it ideal for communications and cryptographic encoding.”
Reid and her colleagues have recently shown how the spooky steering entanglement effect can be shared among many observers, while also enabling extra security even in the presence of hacked devices, which could ultimately form the basis for a future hack-proof ‘quantum internet’.
Quantum teleportation is another application of steering entanglement that could eventually provide a secure ‘facsimile’ capability.
“In quantum mechanics one cannot clone or copy states without making the state fuzzy,” says Reid. Instead, the state is ‘teleported’ between two agents — measurement of the source bit destroys it, and allows the bit to be reinstated exactly and instantly by its spookily entangled partner at any distance.
Quantum simulations
Drummond’s research looks at the deep computational issues arising from the question of how to model and predict the behaviour of quantum systems. With quantum computers still in very early infancy, modelling a quantum system remains a task to be completed on digital computers — an endeavour deemed completely infeasible until recently. “Google’s new quantum hardware is great for certain specialised optimisation problems, but the development of more general quantum hardware is still in its very early stages,” says Drummond. “The software for quantum simulation my group has developed for use on digital computers outperforms all current quantum hardware for quantum simulations.”
The mathematical and computational techniques employed by Drummond’s group invoke a special type of probabilistic simulation called a phase-space representation, which can be calculated in classical terms. “We are able to simulate many of the most exotic quantum states known using these methods, including the idea of creating steering and Schrödinger cats with trapped atoms. This is well beyond anything carried out using quantum computers, and our results are well tested and verified in the laboratory.”
This calculation scheme has allowed Drummond and his team to simulate a Schrödinger cat — a large quantum state consisting of a system of 60 quantum bits, the basic data unit used in quantum computing. “This is important in understanding how one could design and engineer quantum technology for a given task, or even to work out what quantum theory predicts, in order to test it,” notes Drummond.
Coherence is key
Key to realising such applications, however, is maintaining the entanglement state, known in quantum parlance as coherence, over sufficiently long time frames.
Coherence is a touchy thing, easily disrupted or destroyed, particularly for larger particles, and more often than not measured in fractions of a millisecond. “Entanglement can be downgraded by interactions with the environment,” says Reid of the challenge of maintaining coherence.
The natural jitter of atoms at temperatures above absolute zero, for example, is a major source of decoherence, but the Centre for Quantum and Optical Science has made significant progress in dealing with this thermal noise. “Here in the lab, we have atom interferometers in which atoms are ‘frozen’ by laser cooling, which stops the oscillating motion of the atom,” says Reid. “These interferometers are capable of some of the world’s longest decoherence times.”
“We have modelled the full workings of this atom interferometer using our simulation techniques,” says Drummond. He says, the beautiful thing about the entangled atoms we can create using this system is that this is not far away from creating a Schrödinger cat, which would give a rigorous test of the more intriguing predictions of quantum mechanics, such as whether there can be entanglement between two larger, more massive quantum objects.
“We also think we can use the atom interferometer to model and test the quantum field theory of the early universe, which would be very exciting indeed.”