Fast radio bursts could help us estimate the weight of the universe
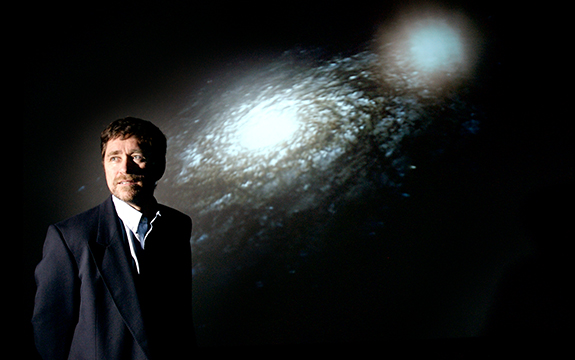
In Summary
- This article originally featured in Swinburne’s Venture magazine.
Being able to ‘weigh’ all the normal matter in something as mind-bogglingly vast as the universe might seem impossible, had it not been for one of those striking, serendipitous discoveries – a brilliant, inexplicable flash of energy from 10 billion years ago. One wild outburst, lasting just five thousandths of a second, that never repeated.
Its discovery was reported to the world back in 2007 by Duncan Lorimer and Swinburne’s then head of astrophysics, Matthew Bailes, and has since prompted furious debate in the astrophysics community about the source of this unheralded phenomenon. But its discovery has opened up an even more intriguing possibility, says Professor Bailes, now Swinburne’s Pro Vice-Chancellor (Research). It may enable us to ‘count’ the intervening electrons between us and the flash, as they interfere with it on its journey across half the universe to us.
If we can count the intervening electrons, says Professor Bailes, we can make a reasonable inference about the amount of normal or baryonic matter lying between us and the flash – and from this reach an educated estimate of what the normal matter in the whole universe actually weighs. (This estimate may, or may not, include the mysterious dark matter – we can’t be sure as yet, as its composition remains unknown. Indeed this approach could shed new light on that issue too.)
To have confidence in such a calculation, what is most needed is a whole family of ‘Lorimer bursts’ – as the phenomenon has been dubbed – reaching us from different directions and identified with galaxies of known distance, so yielding lots of measurements of the average density of the cosmos lying between them and us.
But how do you find something so transient and so rare in such a vast search field? Inspired by the phenomenon, astronomers around the world began a vigorous quest – and drew a blank. Five years later some were saying the burst must have been a one-off, a problem with the instruments, interference from a flash of earthly lighting. Then, in 2012, PhD student Dan Thornton was patiently trawling a relatively sterile region of space on the fringes of the galactic plane looking for needles in the haystack in the form of pulsars and other radio-emitters when, lo and behold, he discerned another, massive flash that faded almost at once.
Putting the pieces together
In the backrooms at Swinburne, the team was ready for it. In the hope the burst would prove to be a repeating event and not a freak, they had quietly been engineering a special piece of equipment capable of visualising it in fine detail. The puzzle, says Professor Bailes, is that there were just these two giant flashes, whereas if they were from a regular, fairly common event, one would expect to see a host of smaller ones, at least.
The second discovery reignited the search. Gazing in a narrow part of the radio spectrum, Thornton went on to detect three more, and by late 2013 up to 10 had been reported by astronomers around the world. Plans are now afoot to refurbish the Molonglo Array near Canberra as a dedicated search instrument for these fast radio bursts (FRBs), as they are now known. And Australia’s giant Square Kilometre Array will augment the hunt, when it comes online. In time enough bursts will be measured to begin the epic calculation of the mass of the universe.
Finding the source of the blasts
As to the cause of the flash, astrophysicists remain divided. Professor Bailes says the scale of the FRB flashes is consistent with massive objects like large neutron stars, small black holes and possibly giant supernovae. However exploding stars are far more common, their blasts last longer and leave more residue than do FRBs, which are “short, clean and rare,” says Professor Bailes. For this reason he considers the likely source is a magnetar giant flare, from near the surface of a neutron star which, typically, emits more energy in a millisecond that our sun does in 300,000 years and is thus capable of generating such powerful flashes. Neutron stars are thought to be flattened spheres that bulge at their equator and, as they age, become more spherical: when they reach a critical radius they may collapse to form a black hole. Such a collapse could account for the massive energy burst – or some other traumatic realignment of the star’s magnetic field.
The work on FRBs capped a year of spectacular discovery and advances for Swinburne’s Centre for Astrophysics and Supercomputing, which included: taking part in a new experiment to use the timing of radio pulsars to limit the gravity waves emitted when supermassive Black Holes collide; the finding of two distant galaxies that reveal the process by which intense bursts of star formation occur (see breakout box); and the highly successful release of the Centre’s new IMAX documentary film Hidden Universe, playing to audiences around the world.
Visualising the universe
This stunning film is the result of a partnership between Australian documentary filmmaker December Media and Swinburne’s Centre for Astrophysics and Supercomputing team who, in a spectacular fusion of science and art, helped create the breathtaking visuals of the universe into which enthralled audiences are drawn.
Professor Bailes traces the origins of the film to his undergraduate days when he was inspired by an animated film on the TV science show, Cosmos. “There have been massive advances in graphic simulation since then – and our knowledge about the universe has advanced greatly too,” he says. The idea was driven by an evident demand from local school children to find out more about the wonders being disclosed by astronomy and this led to a proposal for a movie depicting the story of the universe in lush 3D imagery, produced with the same supercomputer software that astronomers are using to visualise and animate the astonishing cosmic phenomena they are discovering.
“When December Media saw what Swinburne’s multimedia ‘gun animator’ was doing with our scientific material they said ‘That’s awesome – we should make an IMAX film’. I was a bit sceptical at first, but we raised the money and went ahead, using the Swinburne supercomputer to do the rendering. It took six months,” he recalls.
Barely three months after its local release the film had grossed $300,000, was already in the top 10 Australian movies of 2013 and attracting rave reviews that praised its visual imagery, and educational and entertainment value.
As Swinburne’s astronomers and astrophysicists continue their fascinating journey of exploration into space, time and the deep origins of the cosmos, they are generously taking us all along for the ride.
Watch the Hidden Universe 3D - Theatrical trailer.
Galaxies far, far away
The discovery of two 10-billion-year-old galaxies silhouetted against the brilliant light from even more ancient quasars, reveals for the first time the way these galaxies draw in upon themselves huge quantities of gas and dust and chill it down to form the dense nurseries in which stars are born.
“Most of the star formation activity took place in the early universe around ten billion years ago. The difficulty was being able to see what is happening around and within a galaxy at such immense distances,” explains Professor Mike Murphy. He and postdoc Neil Creighton studied these remote objects using a new technique, integral field spectrometry, which essentially reveals their spectra outlined in the blaze of light from an even more distant quasar.
The spectra contain a number of metals – magnesium, iron, chromium and the like – that do not belong to the quasar. Through this method they have been able to visualise not just the galaxies, but also the long tendrils of gas falling into them, suppling the essential fuel for star formation.
Stars, says Professor Murphy, are born in regions of extremely cold, dense gas and do not ‘ignite’ until they gain sufficient mass. The theory is well known, but needed observations to support or contradict it. A second puzzle was to explain how the warm intergalactic gas was chilled down sufficiently to give rise to star formation – and this is what the observations have supplied.
“We see the gas feeding in towards the galaxy from the intergalactic medium and being funnelled down in long filaments and knots. As these become more dense they cool and so provide the correct medium for star formation,” he explains. “If the gas just contracted in an even spherical pattern onto the galaxy, it would not become cold or dense enough to form stars.
“It’s been a real challenge – but we have now been able to see the basics of the process by which stars are born.”
It’s been a real challenge – but we have now been able to see the basics of the process by which stars are born.